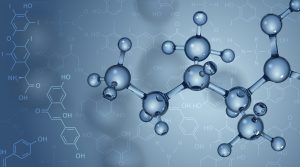
WE CAN HELP YOU!
Contact us NOW for sales & expert advice.
Exclusive Updates
|
Microscope objective lenses, vital optical elements in microscopy, enable precise observation of specimens. Objective lens manufacturers offer a wide range of objective designs for specific needs: high power for detailed observation, scanning for broader views, oil immersion for high-resolution imaging, and long working distance for manipulation without compromising quality. Those objectives are designed with advanced construction techniques for high performance objectives with a spring loaded retractable nose cone assembly that protects the front lens elements and the specimen from collision damage.
Adding to these features, long working distance objectives allow ample space between the lens and the specimen, facilitating the manipulation of samples without compromising image quality. Infinity correction objectives utilize infinity-corrected optical systems, providing flexibility and compatibility with various microscopy accessories.
Numerical aperture, magnification, optical tube length, degree of aberration correction, and other important characteristics are typically imprinted or engraved on the external portion of the barrel for easy reference. These specifications help researchers select the appropriate objective for their experiments, ensuring optimal performance and total magnification when combined with the ocular lens. Specifications like numerical aperture and magnification are typically labeled on the barrel for easy reference. These lenses are indispensable in scientific research providing high powered optics essential for research.
In the following content, we delve intensively into the various components and features of microscope objective lenses, exploring their construction, functionality, and specialized designs that enable researchers to gain deeper insights into the microscopic world.
Components of a microscope
A microscope is an optical device designed to magnify the image of an object, enabling details indiscernible to the human eye to be differentiated. A microscope may project the image onto the human eye or onto a camera or video device.
Microscopes are usually complex assemblies that include an array of lenses, filters, polarizers, and beamsplitters. Illumination is arranged to provide enough light for a clear image, and sensors are used to ‘see’ the object.
Although today’s microscopes are usually far more powerful than the microscopes used historically, they are used for much the same purpose: viewing objects that would otherwise be indiscernible to the human eye. Here we’ll start with a basic compound microscope and go on to explore the components and function of larger more complex microscopes. We’ll also take an in-depth look at one of the key parts of a microscope, the objective lens.
In many microscopes, backlight illumination is favored over traditional direct light illumination due to the latter’s tendency to over-saturate the object under inspection. One specific backlight illumination technique employed in microscopy is Koehler illumination. This method involves flooding the object with light from behind using incident light from a source like a light bulb (see Figure 2). Koehler illumination utilizes two convex lenses, the collector lens and the condenser lens(or called field lens) , to ensure even and bright illumination on both the object and image planes. This design prevents imaging the light bulb filament, a common issue with direct light illumination. Backlight illumination is also commonly referred to as brightfield illumination.
For brightfield illumination to be effective, there needs to be a variation in opacity across the object. Without this variation, the illumination creates a dark blur around the object, resulting in an image with relative contrast between the object’s parts and the light source. Typically, brightfield illumination allows clear visualization of each part of the object unless it is extremely transparent. In cases where transparency hinders feature distinction, darkfield illumination becomes useful.
Darkfield illumination directs light rays obliquely onto the object, avoiding direct entry into the objective. Despite this oblique angle, the rays still illuminate the object plane. The resulting darkfield illumination image achieves high contrast between the transparent object and the light source. In a darkfield setup, a light source forms an inverted cone of light that blocks central rays but allows oblique rays to illuminate the object (see Figure 3). This design effectively forces light to illuminate the object without entering the optical system, making darkfield illumination particularly suitable for transparent objects. In contrast, no rays are blocked in a brightfield illumination setup.
Epi-illumination, a third form of illumination employed in microscopy, generates light from above the objective. This setup replaces the need for a Koehler illumination configuration, as both the objective and the epi-illumination source contribute to the illumination process. The compact structure of epi-illumination is a significant advantage, as the objective serves as a primary source for a considerable portion of the illumination. Figure 4 provides a depiction of a frequently used epi-illumination setup, particularly common in fluorescence applications.
Compound Microscope
While a magnifying glass consists of just one lens element and can magnify any element placed within its focal length, a compound lens, by definition, contains multiple lens elements. A relay lens system is used to convey the image of the object to the eye or, in some cases, to camera and video sensors.
A basic compound microscope could consist of just two elements acting in relay, the objective and the eyepiece. The objective relays a real image to the eyepiece, while magnifying that image anywhere from 4-100x. The eyepiece magnifies the real image received typically by another 10x, and conveys a virtual image to the sensor.
There are two major specifications for a microscope: the magnification power and the resolution. The magnification tells us how much larger the image is made to appear. The resolution tells us how far away two points must be to be distinguishable. The smaller the resolution, the larger the resolving power of the microscope. The highest resolution you can get with a light microscope is 0.2 um, but this depends on the quality of both the objective and eyepiece.
Both the objective lens and the eyepiece also contribute to the overall magnification of the system. If an objective lens magnifies the object by 10x and the eyepiece by 2x, the microscope will magnify the object by 20 times. If the microscope lens magnifies the object by 10x and the eyepiece by 10x, the microscope will magnify the object by 100x. This multiplicative relationship is the key to the power of microscopes, and the prime reason they perform so much better than simply magnifying glasses.
In modern microscopes, neither the eyepiece nor the microscope objective is a simple lens. Instead, a combination of carefully chosen optical components work together to create a high quality magnified image. A basic compound microscope can magnify up to about 1000x. If you need higher magnification, you may wish to use an electron microscope, which can magnify up to a million times.
Key Concepts and Specifications
The majority of microscope objective specifications are conveniently displayed on the objective’s body, including information such as the objective design/standard, magnification, numerical aperture, working distance, lens to image distance, and cover slip thickness correction. Refer to Figure 5 for guidance on interpreting microscope objective specifications. This direct placement of specifications on the objective facilitates a clear understanding of its characteristics, a crucial aspect when integrating multiple objectives into an application. Any additional specifications, like focal length, field of view (FOV), and design wavelength, can be readily calculated or obtained from the vendor or manufacturer’s provided specifications.
For more details, read this article.
Type |
Overview |
Applications |
Achromat |
Corrected for chromatic aberration of two wavelengths (red and blue). This is the most common method, but if you focus on the center of the field of view, the periphery becomes blurred, so it is not suitable for inspection photography. |
|
Plan-Achromat |
Corrected for wavelength chromatic aberration and field curvature aberration. It is suitable for inspection photography because it focuses not only on the center of the field of view but also on the periphery, producing a flat image. |
|
Plan Semi Apochromat |
The chromatic aberration of the three wavelengths, with a slight chromatic aberration remaining in the purple, and the curvature of the field have been corrected. Also called fluorite. In terms of performance, it is positioned between the plan achromat objective lens and the plan apochromat objective lens. High Grade type. |
|
Plan-Apochromat |
Chromatic aberration of three wavelengths and field curvature aberration are corrected. It has a large numerical aperture, excellent resolution, and can obtain ideal images. Highest grade type. |
|
Type |
Overview |
|
Achromat |
|
|
Plan-Achromat | Corrected for two-color (red and blue) chromatic aberration and field curvature aberration. It is suitable for photography because it focuses not only on the center of the field of view but also on the periphery, producing a flat image. | |
Fluorite |
Corrected for chromatic aberration of three colors (red, blue, and violet) with minimal remaining aberration in the purple spectrum. This lens type offers improved color accuracy and resolution compared to achromats. | |
Plan Fluorite |
Combines the correction of three-color chromatic aberration (red, blue, and violet) with field curvature aberration correction. This advanced lens design provides exceptional image quality across the entire field of view, making it ideal for precise microscopy applications requiring high resolution and accurate color rendition. | |
Plan Semi Apochromat |
The chromatic aberration of the three colors (red, blue, and violet), with a slight chromatic aberration remaining in the purple, and the curvature of field have been corrected. Also called fluorite. In terms of performance, it is positioned between the plan achromat objective lens and the plan apochromat objective lens. High Grade type. |
Type |
Overview |
Reflective Objective Lenses |
Microscope objective lenses that include two or more mirrors are called this type of objective that typically boasts a long working distance and zero chromatic aberration, and is useful for FTIR spectroscopy applications. |
Refractive Objective Lenses | Microscope objective made of transparent materials like glass. They use refraction, not reflection, to focus light onto the specimen. These lenses consist of multiple lens elements that correct for optical aberrations and ensure high-quality imaging. |
Type |
Overview |
Oil-immersion Objective Lenses |
Most lenses are designed to work with air between the objective and cover glass, a higher numerical aperture can sometimes be achieved with other substances such as special oil with a specific refractive index. |
Water immersion Objectives Lenses | Water is used as the immersion medium between the objective and cover glass. This allows for a higher numerical aperture, improving resolution and image quality in microscopy applications. |
Air immersion Objective Lenses |
Air is used as the medium between the objective and cover glass. They are commonly used in standard microscopy setups and provide good resolution and image clarity. |
Glycerol immersion Objective lenses |
Combines the correction of three-color chromatic aberration (red, blue, and violet) with field curvature aberration correction. This advanced lens design provides exceptional image quality across the entire field of view, making it ideal for precise microscopy applications requiring high resolution and accurate color rendition. |
Avantier’s Line-ups |
Type of Objectives |
Magnification |
Numerical Aperture |
Working Distance (mm) |
Compatible Brands |
Finite Correction Objectives |
Achromatic, Semi-Plan Achromatic, Plan Achromatic | 1X, 4X, 10X, 20X, 40X, 60X, 100X | 0.1 – 1.25 | 0.13 – 15.8 | – |
Infinitely-Corrected Objectives 2.1 Tube Lens FL = 150mm |
Semi-Plan Achromatic | 4X, 10X, 20X, 40X, 60X, 100X | 0.1 – 1.25 | 0.13- 5.02 | – |
Cover Glass Thickness Corrected 2.2 Tube Lens FL = 180mm |
Semi-Plan Achromatic, Plan Achromatic. Inverted Micro, Fluorescent Objective for Laboratory Micro, Plan Semi Apochromatic, Semi-APO Fluorescent | 2X, 4X, 5X, 10X, 20X, 40X, 60X, 100X | 0.1 – 1.25 | 0.12 – 28 | – |
Cover Glass Thickness Corrected 2.3 Tube Lens FL=200mm |
Plan, Apochromatic, Semi-APO Fluorescent, Semi-APO Metallurgical, Semi-Apochromatic, Plan Achromatic | 2X, 4X, 10X, 20X, 40X, 50X, 60X, 100X | 0.1 – 1.45 | 0.13 – 20.6 | – |
Compatible Tube Lens EFL:200mm |
Plan Fluor EPI & EPI BD | 5X, 10X, 20X, 50X, 100X | 0.14 – 0.9 | 1 – 20 | Olympus equivalent |
Polarizing Objectives |
Polarizing | 4X, 10X, 20X, 40X, 100X | 0.1 – 1.25 | 0.2 – 30 | – |
Phase Contrast Objectives |
Plan Phase Contrast, Semi-Apochromatic | 4X, 10X, 20X, 40X, 60X, 100X | 0.2 – 1.25 | 0.2 – 17.2 | – |
Semi-APO Metallurgical Objectives |
Semi-APO Metallurgical | 5X, 10X, 20X, 50X, 100X | 0.15 – 0.9 | 1 – 20 | – |
Flat Field Achromatics Objectives |
Infinitely Conjugated Ultra-Long Working Distance Flat Field Achromatic | 1X, 2X, 3.5X, 5X, 7.5X, 10X, 15X, 20X, 50X | 0.025 – 0.55 | 13 – 41 | – |
Plan ApochromaticObjectives |
Infinitely Far Conjugated Flat Field Achromatic, M Plan Apo NIR, LCD Plan Apo NIR. M Plan Apo NUV (HR) | 5X, 10X, 20X, 50X, 100X | 0.21 – 0.9 | 1.4 – 20 | Mitutoyo equivalen |
Infinitely Conjugated Flat Field Apochromatic Near-Ultraviolet Objective |
M Plan Apo NUV | 20X, 50X, | 0.42 – 0.65 | 10 – 17 | |
Infinite Conjugated Ultra-Long Working Distance Bright Field Objective |
M Plan Apo NIR, i Plan Apo HR, M Plan Apo HR NIR, M Plan Apo SL | 20X, 50X, 100X | 0.42 – 0.75 | 4 – 30 | Mitutoyo equivalent |
Small Objectives (Lens diameter <4mm) |
3X, 7X | 0.45 – 0.7 | 0.01 – 1 |
Microscope objectives are pivotal components in optical microscopy, especially in influencing image quality and resolution. Selecting the right objective is crucial for achieving optimal results in your microscopy applications. To guide you through the selection process, consider the following factors:
Choosing the right microscope objective is pivotal for optimal imaging performance. Consider your specific application requirements, utilize the provided guide, and explore Avantier’s diverse objective offerings to ensure accurate and reliable results in your microscopy endeavors.
Avantier is a premier manufacturer of high performance microscope objective lenses, and we produce a wide range of quality microscope objectives for applications ranging from research to industry to forensics and medical diagnostics. We carry many types of objectives in stock, including apochromat objectives, achromatic objectives, and semi apochromat objectives. We can also produce custom objectives designed to work as desired in your target spectral range.
For custom microscope objective lenses, visit our microscope objective lenses page.
If you’re interested in acquiring in-stock microscope objective lenses, please visit our ‘Stock – Microscope Objective‘ page.
Fluorescence Microscopy
Fluorescence microscopy is a powerful imaging technique used primarily in biomedical research to visualize and study samples labeled with fluorescent dyes or proteins at the microscopic level. The method relies on the phenomenon of fluorescence, where materials absorb light at a specific wavelength (excitation light) and then emit light at a longer wavelength (emission wavelength). A focused light source, such as a laser, is used to selectively excite fluorescent molecules within the sample. The emitted fluorescence is captured to form detailed images, providing valuable information about the sample’s internal structure and composition.
Confocal Microscopy
Confocal microscopy offers the capability to capture sharp images from a slender slice of a dense sample, minimizing background noise and reducing out-of-focus disturbances. Optical sectioning, widely employed in biomedical science and materials science, involves placing a sample on the microscope stage. An image is initially acquired at the primary focal plane, and subsequently, the stage or objective is adjusted vertically to capture images at successive focal planes.
IR Microscope
Infrared microscopy, alternatively referred to as infrared microspectroscopy, is a form of light microscopy that employs a light source transmitting infrared wavelengths to observe a sample’s image. In contrast to conventional optical microscopes utilizing absorbent glass optics, an infrared microscope incorporates reflective optics, enabling it to encompass the complete spectral range of infrared light.
Laser Ablation
Lasers find widespread applications, commonly employed to either (1) heat material onto a base or (2) ablate material off of a base. Laser ablation systems necessitate the integration of microscope components due to the precise manipulation of the laser beam, including focusing, bending, and reducing scattering. Typically, a laser ablation setup incorporates custom optics instead of off-the-shelf components, with the laser intricately designed into the system, as illustrated in Figure 14. The laser is strategically oriented in an epi-illumination design to leverage the microscope objective’s capacity to focus light at the object plane, generating exceptionally small spot sizes with minimal aberrations. Additionally, an eyepiece enables the user to visually locate the laser and ensure proper functionality. Filters are indispensable in shielding the user’s eyes from potential laser damage. Laser ablation setups, known for their superior precision compared to traditional surgical methods, find applications in medical and biological contexts.
Related Content
Contact us NOW for sales & expert advice.